A signal challenge for the mother cell.
A signal from the spore cell facilitates the expression of late genes in the mother cell of Bacillus subtilis during sporulation.
S. Vickery.
Summary
Endospore formation in Bacillus subtilis is induced by envrironmental pressures such as limited nutrients or a deviation of pH from the permissible range, which pose a threat to the organism. (Prince et al., 2005). Sporulation results in death of the mother cell allowing release of a resistant spore into the environment, which greatly increases the probability of that cell's survival in extreme conditions. Following the detection of an environmental threat, the cell proceeds through a series of morphological changes, collectively called 'sporulation' which results in the production and consequent release of the developed spore cell. Firstly, DNA is replicated and the two chromosomes are directed to opposite poles by an axial filament. Secondly, a septum is formed close to one pole of the cell, resulting in one large and one small cellular compartment known as the mother cell and forespore respectively (Stragier and Losick, 1996). The mother cell then engulfs the forespore, which becomes a free endospore cell within the mother cell (Stragier and Losick, 1996).
In the figure:

Figure 1. The morphological stages of sporulation. Source: Stragier and Losick, 1996. (Original Figure was unavailable. An alternative from the net at http://www.ibpc.fr/UPR9073/psfr.html was substituted)
During sporulation in Bacillus subtilis, the forespore and the mother cell follow different patterns of gene expression as defined by the presence of unique sigma factors that are confined to either cell (Stragier and Losick, 1996; Zhang et al., 1998). σF and σG are present only in the forespore while σE and σK are present exclusively in the mother cell (Rudner and Losick, 2002). These sigma factors induce cell-specific gene expression and account for the varying morphological and physiological requirements in the two cells. For example, a regulon of late genes under the control of σK, is expressed exclusively in the mother cell and facilitates three key processes; spore coat biosynthesis, final spore maturation and lysis of the mother cell via apoptosis to implement release of the spore cell (Zhang et al., 1998). The activation of these late genes in the mother cell is a particularly well-studied and intriguing example of the complex interactions that occur between the mother cell and forespore throughout sporulation, via intercellular signalling.
The cascade of signalling events required to activate sigma factors, and thus induce gene expression in the two cells, ensures that sporulation is tightly regulated and is maintained in an orderly sequential process. The sigma factors in both the mother cell and the forespore cell exist in an inactive form until the cells receive signals from each other, allowing the sigma factors to be activated, thus inducing the expression of a specific set of genes (Rudner and Losick, 2002; Stragier and Losick, 1996; Wakeley et al., 2000). In the case of pro-σK in the mother cell, it is the forespore-derived protein SpoIVB that acts on components in the mother cell to mediate proteolytic cleavage of pro-σK to active σK (Zhang et al., 1998; Dong and Cutting 2003; 2004; Wakeley et al., 2000).
SpoIVB does not directly process pro-σK to its active state. Rather, it relieves an inhibitory effect that is imposed upon SpoIVFB enzyme in the mother cell which functions to process pro-σK (Zhang et al., 1998; Rudner and Losick, 2002; Wakeley et al., 2000, Dong and Cutting, 2003). SpoIVFB enzyme is maintained in an inactive form by BofA protein in the mother cell until the SpoIVB signal is received from the spore cell (Zhang et al., 1998). This is made possible by the presence of an additional protein in the mother cell (SpoIVFA) which plays a central role in BofA's inhibitory function. Research to date supports a model whereby SpoIVFA facilitates the interaction between BofA and SpoIVFB by serving as a platform to draw these two proteins into proximity to one another (Rudner and Losick, 2002). Only once BofA and SpoIVFB are adjacent can BofA impose its inhibitory effect upon SpoIVFB (Zhang et al., 1998; Rudner and Losick, 2002). A multimeric complex is formed between the three mother cell proteins and embeds in the mother-cell membrane that surrounds the forespore, permeating into the intermembrane space, which is where it interacts with SpoIVB from the forespore (Zhang et al., 1998). The subcellular localisation of the SpoIVFB-SpoIVFA-BofA complex is thought to take place via a conserved domain in SpoIVFA which interacts with peptidoglycan in the intermembrane space between the mother and forespore cells (Zhang et al., 1998).
Fig.2. Proteolytic processing of pro-σK in the mother cell is catalyzed by SpoIVfB which exists in complex with its two regulators, SpoIVFA and BofA, until SpoIVB activates processing by reversing the inhibition imposed on SpoIVFB by BofA. Source: Rudner and Losick, 2002.
SpoIVB enzyme produced in the forespore is able to relieve an inhibitory event in the mother cell by interacting with the pro-σK complex in intermembrane space. SpoIVB crosses the forespore membrane to reside in the intermembrane space (Wakeley et al., 2000). The PDZ domain in SpoIVB recognises the COOH terminus of another SpoIVB molecule in the forespore (Dong and Cutting, 2004). The interaction between the two SpoIVB molecules activates their cleavage into a form that can be released across the spore cell membrane into the intermembrane space. The knowledge that SpoIVB can move across a phospholipid bilayer in Escherichia coli invoked the suggestion that it may also cross a membrane in this system, which was consequently proved by experiment (Wakeley et al., 2000).
Once in the intermembrane space, SpoIVB is able to induce the activation of pro-σK, made possible by the fact that the pro-σK initiating complex is conveniently embedded in the mother cell membrane surrounding the forespore awaiting a signal from the forespore (Dong and Cutting 2004). SpoIVB undergoes a second cleavage event; autoproteolysis, to produce short protein fragments which interact with the pro-σK initiation complex inducing pro-σK processing (Wakeley et al., 2000). This cleavage event is a critical aspect of this protein's ability to signal pro-σK processing, as demonstrated through examination of spoIVB gene mutants in which autoproteolysis was blocked resulting in failed activation of pro-σK processing (Wakeley et al., 2000).
The PDZ domain in the active products resulting from the second cleavage event target the pro-σK processing complex embedded in the mother cell membrane surrounding the forespore. SpoIVB binds the COOH terminus of BofA in the pro-σK initiating complex which draws SpoIVB into proximity to SpoIVFA. Using its PDZ domain, SpoIVB recognises an internal motif in SpoIVFA and associates with it, inducing cleavage of SpoIVFA and subsequent dissassemby of the multimeric complex (Stragier and Losick, 1996). SpoIVFA releases BofA and SpoIVFB from its clasp once it is cleaved, so BofA can no longer inhibit the enzymatic action of SpoIVFB. SpoIVFB is then available to process pro-σK to σK. As a result of interaction with SpoIVB, SpoIVFB is released from its inhibited state in the complex and is available to activate proteolytic processing of pro-σK to produce the active σK. This occurs at the mother cell membrane into which inactive pro-σk has also embedded, proximal to the multimeric complex (Rudner and Losick, 2002; Wakeley et al., 2000; Zhang et al., 1998). Presumably the migratory mechanism employed by pro-σk to reside in the mother cell membrane has evolved to maximise the efficiency of its processing as it enables pro-σK to be present when SpoIVFB is activated.

Figure 3. SpoIVB protein undergoes at least 3 cleavage events.
A third and final cleavage event inactivates SpoIVB (Dong and Cutting 2003; 2004). This series of cleavage events is heavily reliant on the presence of a PDZ domain in the SpoIVB protein, as defined by experiments in which SpoIVB proteins carrying various PDZ mutations had impaired cleavage ability (Dong and Cutting 2003).
The signal communicated by SpoIVB from the forespore induces a cascade of proteolytic cleavage events in the mother cell that enable pro-σK to be processed and the late genes under σK control to be expressed (Stragier and Losick, 1996). The interaction between SpoIVB and SpoIVFA in the intermembrane space between the spore cell and mother cell is where products from the two cells meet. It is by way of these proteins intersecting that the spatially discrete cells can communicate despite the restrictive presence of two cellular membranes. This is achieved by employment of a complex pathway through which SpoIVB targets SpoIVFA to overcome its inhibitory effect upon SpoIVFB. As this example of signal transduction involves the signalling protein, SpoIVB, acting across a cell membrane, this system offers an important insight into the mechanisms at work in intercompartmental signalling. In particular, that this system of transmembrane signalling essentially relies upon the PDZ domains recognising DNA motifs, and regulated proteolysis. This defines the mechanism of intercompartmental signalling by which SpoIVB induces pro-σk processing.
In order to further expand upon the knowledge of how pro-σK processing pathway operates, it would be useful to understand the interaction between BofA and SpoIVFB proteins in the mother cell in more detail. It is known that BofA is most certainly responsible for inhibiting SpoIVFB, but the current models surrounding an interaction between the two proteins are based on speculation. It is known that SpoIVFA draws these two proteins together, and the current models favour BofA and SpoIVFB directly interacting but the more complicated model has not yet been ruled out, whereby BofA induces a confomational change in SpoIVFA, the altered state of which is responsible for inhibiting SpoIVFB enzyme (Rudner and Losick, 2002).
Also, further investigation of the function of SpoIVB protein from the spore cell would provide greater insight into the process of sporulation in Bacillus subtilis. Observation of cells carrying a spoIVB null mutation imply that this protein is not only active in pro-σK processing. In spoIVB null mutants pro-σK processing is constitutively active, but cells fail to make intact heat-resistant spores, so SpoIVB protein must have a second, as yet undefined role in spore formation (Dong and Cutting, 2004)
References
T.C. Dong and S.M. Cutting (2004) The PDZ domain of the SpoIVB transmembrane signalling protein enables cis-trans interactions involving multiple partners leading to the activation of the pro-σK processing complex in Bacillus subtilis. The Journal of Biological Chemistry 279(42): 43468-43478.
T.C. Dong and S.M. Cutting (2003) SpoIVB-mediated cleavage of SpoIVFA could provide the intercellular signal to activate processing of pro-σK in Bacillus subtilis. Molecular Microbiology 49(5): 1425-1434.
D.Z. Rudner and R. Losick (2002) A sporulation membrane protein tethers the pro-sk processing enzyme to its inhibitor and dictates its subcellular localization. Genes and Development 16: 1007-1018.
P.R. Wakeley, R. Dorazi, N. Thi Hoa, J.R. Bowyer and S.M. Cutting (2000) Proteolysis of SpoIVB is a critical determinant in signalling of pro-σK processing in Bacillus subtilis. Molecular Microbiology 36(6): 1336-1348.
B. Zhang, A. Hofmeister and L. Kroos (1998) The prosequence of pro-σK promotes membrane association and inhibits RNA polymerase core binding. Journal of Bacteriology 180(9): 2434-2441.
P. Stragier and R. Losick (1996) Molecular genetics of sporulation in Bacillus Subtilis. Annual Review of Genetics 30: 297-341.
H. Prince, R. Zhou and L. Kroos (2005) Substrate requirements for regulated intramembrane proteolysis of Bacillus subtilis pro-σK. Journal of Bacteriology 187: 961-971.
S. Vickery.
Summary
During sporulation in Bacillus subtilis, a late regulon of genes under control of sigma factor K must be expressed in the mother cell to implement the final maturation and release of the spore cell. SpoIVB protein is necessary for intercompartmental signalling between the forespore and mother cell, resulting in activation of precursor pro-σK to active σK. This is achieved via a complex pathway through which SpoIVB protein, produced in the spore cell, traverses through the forespore cell membrane into the intermembrane space where it acts on components from the mother cell to mediate pro-σK processing. SpoIVB acts to overcome an inhibitory effect upon SpoIVFB enzyme in the mother cell, which cleaves pro-σK to σK. SpoIVFA protein draws together and tethers SpoIVFB and BofA proteins in the mother cell, allowing BofA to impose an inhibitory effect upon SpoIVFB. The SpoIVB signal from the spore cell cleaves SpoIVFA, thus disengaging SpoIVFB and BofA, enabling SpoIVFB to activate pro-σK.
Endospore formation in Bacillus subtilis is induced by envrironmental pressures such as limited nutrients or a deviation of pH from the permissible range, which pose a threat to the organism. (Prince et al., 2005). Sporulation results in death of the mother cell allowing release of a resistant spore into the environment, which greatly increases the probability of that cell's survival in extreme conditions. Following the detection of an environmental threat, the cell proceeds through a series of morphological changes, collectively called 'sporulation' which results in the production and consequent release of the developed spore cell. Firstly, DNA is replicated and the two chromosomes are directed to opposite poles by an axial filament. Secondly, a septum is formed close to one pole of the cell, resulting in one large and one small cellular compartment known as the mother cell and forespore respectively (Stragier and Losick, 1996). The mother cell then engulfs the forespore, which becomes a free endospore cell within the mother cell (Stragier and Losick, 1996).
In the figure:
- An axial filament directs the separation of chromosomes
- A septum is formed
- The mother cell engulfs the spore cell
- The mother cell is lysed and the spore cell is released.

Figure 1. The morphological stages of sporulation. Source: Stragier and Losick, 1996. (Original Figure was unavailable. An alternative from the net at http://www.ibpc.fr/UPR9073/psfr.html was substituted)
During sporulation in Bacillus subtilis, the forespore and the mother cell follow different patterns of gene expression as defined by the presence of unique sigma factors that are confined to either cell (Stragier and Losick, 1996; Zhang et al., 1998). σF and σG are present only in the forespore while σE and σK are present exclusively in the mother cell (Rudner and Losick, 2002). These sigma factors induce cell-specific gene expression and account for the varying morphological and physiological requirements in the two cells. For example, a regulon of late genes under the control of σK, is expressed exclusively in the mother cell and facilitates three key processes; spore coat biosynthesis, final spore maturation and lysis of the mother cell via apoptosis to implement release of the spore cell (Zhang et al., 1998). The activation of these late genes in the mother cell is a particularly well-studied and intriguing example of the complex interactions that occur between the mother cell and forespore throughout sporulation, via intercellular signalling.
The cascade of signalling events required to activate sigma factors, and thus induce gene expression in the two cells, ensures that sporulation is tightly regulated and is maintained in an orderly sequential process. The sigma factors in both the mother cell and the forespore cell exist in an inactive form until the cells receive signals from each other, allowing the sigma factors to be activated, thus inducing the expression of a specific set of genes (Rudner and Losick, 2002; Stragier and Losick, 1996; Wakeley et al., 2000). In the case of pro-σK in the mother cell, it is the forespore-derived protein SpoIVB that acts on components in the mother cell to mediate proteolytic cleavage of pro-σK to active σK (Zhang et al., 1998; Dong and Cutting 2003; 2004; Wakeley et al., 2000).
SpoIVB does not directly process pro-σK to its active state. Rather, it relieves an inhibitory effect that is imposed upon SpoIVFB enzyme in the mother cell which functions to process pro-σK (Zhang et al., 1998; Rudner and Losick, 2002; Wakeley et al., 2000, Dong and Cutting, 2003). SpoIVFB enzyme is maintained in an inactive form by BofA protein in the mother cell until the SpoIVB signal is received from the spore cell (Zhang et al., 1998). This is made possible by the presence of an additional protein in the mother cell (SpoIVFA) which plays a central role in BofA's inhibitory function. Research to date supports a model whereby SpoIVFA facilitates the interaction between BofA and SpoIVFB by serving as a platform to draw these two proteins into proximity to one another (Rudner and Losick, 2002). Only once BofA and SpoIVFB are adjacent can BofA impose its inhibitory effect upon SpoIVFB (Zhang et al., 1998; Rudner and Losick, 2002). A multimeric complex is formed between the three mother cell proteins and embeds in the mother-cell membrane that surrounds the forespore, permeating into the intermembrane space, which is where it interacts with SpoIVB from the forespore (Zhang et al., 1998). The subcellular localisation of the SpoIVFB-SpoIVFA-BofA complex is thought to take place via a conserved domain in SpoIVFA which interacts with peptidoglycan in the intermembrane space between the mother and forespore cells (Zhang et al., 1998).
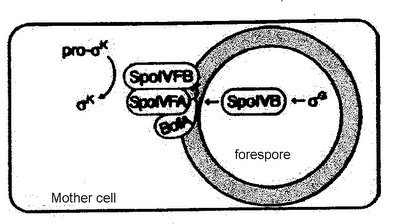
SpoIVB enzyme produced in the forespore is able to relieve an inhibitory event in the mother cell by interacting with the pro-σK complex in intermembrane space. SpoIVB crosses the forespore membrane to reside in the intermembrane space (Wakeley et al., 2000). The PDZ domain in SpoIVB recognises the COOH terminus of another SpoIVB molecule in the forespore (Dong and Cutting, 2004). The interaction between the two SpoIVB molecules activates their cleavage into a form that can be released across the spore cell membrane into the intermembrane space. The knowledge that SpoIVB can move across a phospholipid bilayer in Escherichia coli invoked the suggestion that it may also cross a membrane in this system, which was consequently proved by experiment (Wakeley et al., 2000).
Once in the intermembrane space, SpoIVB is able to induce the activation of pro-σK, made possible by the fact that the pro-σK initiating complex is conveniently embedded in the mother cell membrane surrounding the forespore awaiting a signal from the forespore (Dong and Cutting 2004). SpoIVB undergoes a second cleavage event; autoproteolysis, to produce short protein fragments which interact with the pro-σK initiation complex inducing pro-σK processing (Wakeley et al., 2000). This cleavage event is a critical aspect of this protein's ability to signal pro-σK processing, as demonstrated through examination of spoIVB gene mutants in which autoproteolysis was blocked resulting in failed activation of pro-σK processing (Wakeley et al., 2000).
The PDZ domain in the active products resulting from the second cleavage event target the pro-σK processing complex embedded in the mother cell membrane surrounding the forespore. SpoIVB binds the COOH terminus of BofA in the pro-σK initiating complex which draws SpoIVB into proximity to SpoIVFA. Using its PDZ domain, SpoIVB recognises an internal motif in SpoIVFA and associates with it, inducing cleavage of SpoIVFA and subsequent dissassemby of the multimeric complex (Stragier and Losick, 1996). SpoIVFA releases BofA and SpoIVFB from its clasp once it is cleaved, so BofA can no longer inhibit the enzymatic action of SpoIVFB. SpoIVFB is then available to process pro-σK to σK. As a result of interaction with SpoIVB, SpoIVFB is released from its inhibited state in the complex and is available to activate proteolytic processing of pro-σK to produce the active σK. This occurs at the mother cell membrane into which inactive pro-σk has also embedded, proximal to the multimeric complex (Rudner and Losick, 2002; Wakeley et al., 2000; Zhang et al., 1998). Presumably the migratory mechanism employed by pro-σk to reside in the mother cell membrane has evolved to maximise the efficiency of its processing as it enables pro-σK to be present when SpoIVFB is activated.

Figure 3. SpoIVB protein undergoes at least 3 cleavage events.
- 1. The PDZ domain of SpoIVB recognising the COOH terminus of another SpoIVB molecule prompting cleavage which releases the molecules into the intermembrane space.
- 2. Autoproteolysis of SpoIVB produces an active protein fragment which targets the Pro-sigmaK signalling complex.
- 3. Further cleavage occurs to inactivate SpoIVB.
A third and final cleavage event inactivates SpoIVB (Dong and Cutting 2003; 2004). This series of cleavage events is heavily reliant on the presence of a PDZ domain in the SpoIVB protein, as defined by experiments in which SpoIVB proteins carrying various PDZ mutations had impaired cleavage ability (Dong and Cutting 2003).
The signal communicated by SpoIVB from the forespore induces a cascade of proteolytic cleavage events in the mother cell that enable pro-σK to be processed and the late genes under σK control to be expressed (Stragier and Losick, 1996). The interaction between SpoIVB and SpoIVFA in the intermembrane space between the spore cell and mother cell is where products from the two cells meet. It is by way of these proteins intersecting that the spatially discrete cells can communicate despite the restrictive presence of two cellular membranes. This is achieved by employment of a complex pathway through which SpoIVB targets SpoIVFA to overcome its inhibitory effect upon SpoIVFB. As this example of signal transduction involves the signalling protein, SpoIVB, acting across a cell membrane, this system offers an important insight into the mechanisms at work in intercompartmental signalling. In particular, that this system of transmembrane signalling essentially relies upon the PDZ domains recognising DNA motifs, and regulated proteolysis. This defines the mechanism of intercompartmental signalling by which SpoIVB induces pro-σk processing.
In order to further expand upon the knowledge of how pro-σK processing pathway operates, it would be useful to understand the interaction between BofA and SpoIVFB proteins in the mother cell in more detail. It is known that BofA is most certainly responsible for inhibiting SpoIVFB, but the current models surrounding an interaction between the two proteins are based on speculation. It is known that SpoIVFA draws these two proteins together, and the current models favour BofA and SpoIVFB directly interacting but the more complicated model has not yet been ruled out, whereby BofA induces a confomational change in SpoIVFA, the altered state of which is responsible for inhibiting SpoIVFB enzyme (Rudner and Losick, 2002).
Also, further investigation of the function of SpoIVB protein from the spore cell would provide greater insight into the process of sporulation in Bacillus subtilis. Observation of cells carrying a spoIVB null mutation imply that this protein is not only active in pro-σK processing. In spoIVB null mutants pro-σK processing is constitutively active, but cells fail to make intact heat-resistant spores, so SpoIVB protein must have a second, as yet undefined role in spore formation (Dong and Cutting, 2004)
References
T.C. Dong and S.M. Cutting (2004) The PDZ domain of the SpoIVB transmembrane signalling protein enables cis-trans interactions involving multiple partners leading to the activation of the pro-σK processing complex in Bacillus subtilis. The Journal of Biological Chemistry 279(42): 43468-43478.
T.C. Dong and S.M. Cutting (2003) SpoIVB-mediated cleavage of SpoIVFA could provide the intercellular signal to activate processing of pro-σK in Bacillus subtilis. Molecular Microbiology 49(5): 1425-1434.
D.Z. Rudner and R. Losick (2002) A sporulation membrane protein tethers the pro-sk processing enzyme to its inhibitor and dictates its subcellular localization. Genes and Development 16: 1007-1018.
P.R. Wakeley, R. Dorazi, N. Thi Hoa, J.R. Bowyer and S.M. Cutting (2000) Proteolysis of SpoIVB is a critical determinant in signalling of pro-σK processing in Bacillus subtilis. Molecular Microbiology 36(6): 1336-1348.
B. Zhang, A. Hofmeister and L. Kroos (1998) The prosequence of pro-σK promotes membrane association and inhibits RNA polymerase core binding. Journal of Bacteriology 180(9): 2434-2441.
P. Stragier and R. Losick (1996) Molecular genetics of sporulation in Bacillus Subtilis. Annual Review of Genetics 30: 297-341.
H. Prince, R. Zhou and L. Kroos (2005) Substrate requirements for regulated intramembrane proteolysis of Bacillus subtilis pro-σK. Journal of Bacteriology 187: 961-971.
0 Comments:
Post a Comment
<< Home